This was an investigation that I did in 1986 (Note 1). It was prompted by my interest in early cements, having read Major Francis’ book, and in particular his discussion of Roman cements (Note 2), and follow-up reading of the lectures by Dr Halstead (Note 3) and Professor Skempton (Note 4). It becomes apparent that there are question marks over the analytical data quoted and the phases calculated in the compositions of the various historic building materials of the first half of the nineteenth century. This coincided with the recent arrival of a high-temperature furnace at Shoreham, where I was working at the time, and the availability of relatively precise XRF analysis methods. The analyses, although good by British standards, were subsequently (1995) repeated at Atlanta, where a system with state-of-the-art accuracy and precision was available.
In this study, the effects of uncertainty about particle size and inhomogeneity of raw materials was avoided by deliberately selecting a highly combinable pair of components, and making all mixes from these. The two components were both obtained from Pitstone (Note 5).
- The high-Ca (“chalk”) component was from the “white pit” and was a fine-grained flint-free Middle Chalk with a moisture content of 15.9%. About a kilogram was wet-ground in 50 g lots with 25 mL water. The ground material was placed on a 90-μm sieve and washed through. Material retained on the sieve (typically less than 0.5 g) was discarded. The combined suspension of fines was allowed to settle over a period of several weeks, the supernatant water was decanted, and the remaining slurry was removed, leaving a small layer of coarser material at the base. The slurry was dried at 110ºC and the resulting “biscuit” material was rubbed through a 90-μm sieve. This yielded about 750 g of fine white powder with the following sieve size distribution:
Sieve Size μm % retained 90 0 75 0.8 63 2.0 53 3.7 45 5.8 - The low-Ca (“clay”) component was from the base of No 3 Marl pit, and was a fine-grained Chalk Marl with a moisture content of 18.7%. It had a CaCO3 content of around 71%. About 2 kg of this material was rough-crushed and dried, and crushed again to around 2 mm. This was treated, in 100 g lots, with 1 L of water followed by slow addition of 150 mL concentrated hydrochloric acid. The combined suspensions were allowed to settle for several weeks, the supernatant liquid was decanted, and the residue, neglecting a small amount of gritty material at the bottom, was filtered and washed with water until chloride-free. It was then washed through a 45-μm sieve with water, again settled, filtered, and washed with propan-2-ol then dried at 110 ºC. This yielded about 310 g of fine chocolate-brown powder, all under 45 μm.
These materials were combined in various proportions in twenty 40 g lots: the powders were slurried with 30 mL water, well mixed and dried. The dried material was roughly hand-ground and re-mixed, then pressed at 200 kN into a cylindrical pellet of dimensions 40 mm diameter by about 11 mm thick. The pellet, in a 50 mm platinum dish, was placed in the furnace set at 600ºC, and the temperature was raised at 15ºC per minute (Note 6) to either 1150ºC or 1380ºC. The lower target temperature was reached in 45 minutes and the higher in 88 minutes. In both cases total furnace time was 120 minutes, after which the crucible was removed and rapidly cooled by suspension in water, seated in a splash guard. On no occasion was there any indication of “dusting” (Note 7), although the pellets, intact up to that point, usually warped and cracked into a few large pieces on cooling. The material was rapidly hand ground to <100 μm and free lime was determined by selective solution in ethane-1,2-diol. Phases were then calculated, using Taylor’s phase compositions (Note 8).
The chemical analyses of the two components (ASTM C 114, revised 1995) were:
Analyte | Chalk | Clay |
---|---|---|
SiO2 | 0.84 | 66.37 |
Al2O3 | 0.15 | 17.01 |
Fe2O3 | 0.10 | 5.87 |
CaO | 54.68 | 1.44 |
MgO | 0.56 | 1.33 |
SO3 | 0.06 | 0.57 |
LoI950 | 43.51 | 3.82 |
Na2O | 0.005 | 0.48 |
K2O | 0.017 | 1.74 |
SrO | 0.065 | 0.01 |
TiO2 | 0.008 | 0.83 |
P2O5 | 0.004 | 0.41 |
Mn2O3 | 0.023 | 0.13 |
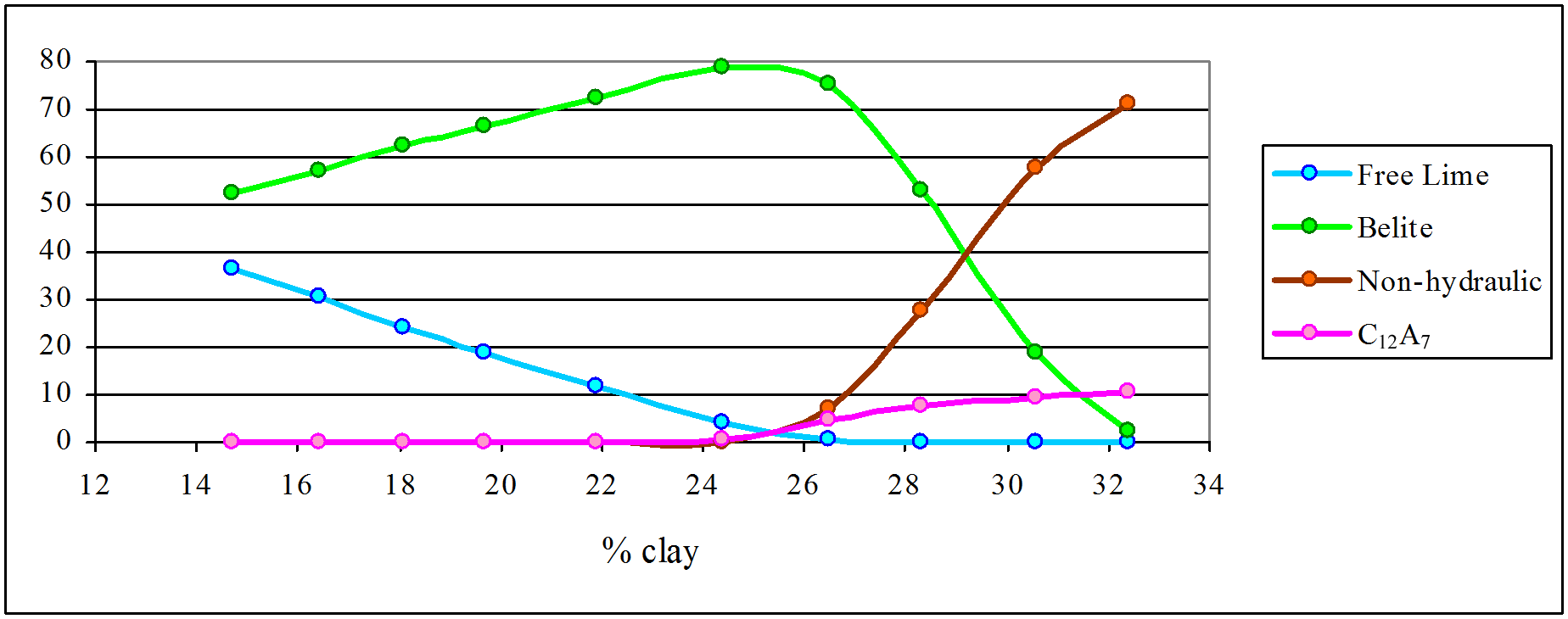
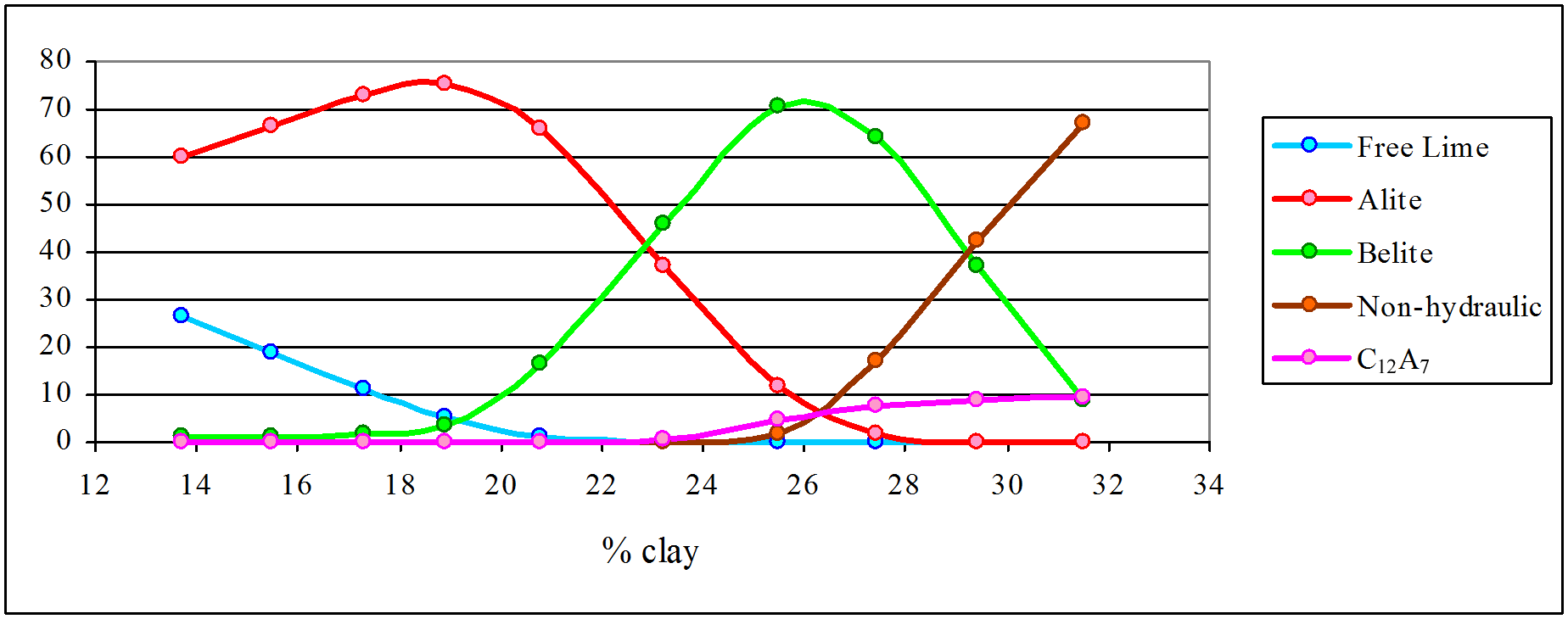
The lower temperature series shows the break between hydraulic limes and “Roman” cement at around 25-26% clay content. The hydraulic limes lack reactive aluminates and are slow setting. In the range 19-25% clay content, hydraulic limes have less than 20% free lime. These would slake reluctantly and need to be ground prior to use: they correspond to Vicat’s typical product. Among the “Roman” cements, rapid set is brought about by rising content of reactive aluminates, but belite content falls rapidly with rising clay content, and probably become non-viable above 30% clay content.
The higher temperature series yields only Portland cement as a viable product, with clay contents between 19 and 25%. The lower limit is governed by the free lime content. Below 19% clay content, we have a mixture of alite and dead-burned lime which develops strength rapidly, but becomes unsound. Above 25% clay, alite (which characterises Portland cement) disappears and the product becomes indistinguishable from that of “Roman” cement, although because of the high temperature, the product vitrifies. It is because of having to grind this hard material, and the waste of kiln fuel, that “Roman” cement manufacturers avoided higher temperatures. It is quite incorrect to suggest that, had they ground their discarded clinker, “Roman” cement manufacturers would have “discovered” Portland cement, since their clay content was outside the Portland range. On the other hand, manufacturers of artificial hydraulic limes of the Vicat type – and there were none of these in Britain – might well have done so.
The last point also disproves the frequently-repeated error, that Joseph Aspdin “discovered” modern Portland cement simply by burning harder. The innovation also involved a deliberate increase in lime content, and can’t be seen as accidental. This fits with the narrative of the product’s invention by William Aspdin.