I'm a chemist (Note 1). As a child - say, under 12 years old - I was interested in everything. History, geography, mathematics, art, science; in science probably mainly the many aspects of physics. I was aware of chemistry as a somewhat niche area. Why did I start to think of chemistry as my "special subject"?
I was born and raised in Luton, Bedfordshire, in the chalky downlands of the Chilterns. Luton was then a dynamic and prosperous industrial town, and I was raised in the aristocracy of its working class. "Aristocracy". Is that excessive? Let me explain. My primary education was in a very working-class area, largely full of council housing, and my school friends were entirely from there. I remember being mildly struck by the fact that my working-class friends' houses contained very few books. Later, I went to Luton Grammar School, and acquired much more well-heeled middle-class friends. Their houses, I noticed, contained only a token number of not-very-well-thumbed books. My house, by contrast, was full of books, from floor to ceiling, in every room. This was a phenomenon only to be found among my family's acquaintances, consisting of Trade Union officials, left-wing activists and American refugees (this being the McCarthy era) (Note 2).
When he was de-mobbed after WWII, my father bought a remaindered set of Encyclopaedia Britannica, presumably rendered wildly out-of-date by the events of the war. I spent many an hour reading through the 24 volumes (or just looking at the pictures). I particularly liked Volume 4. Chemistry was in Volume 5. Widely diverse subjects rubbed shoulders in the strict alphabetical order. You look up Glaciers and end up finding all about Gladiators and Gladstone. Each article was written by a world-expert on its subject (an idea entirely foreign to users of Wikipedia). By the time I had passed my eleven-plus and moved to the Grammar School, I was as much a polymath as any 12-year-old could claim to be.
In the second year of Grammar School, in class 2A, we first entered the reasonably well-appointed chemistry lab. I had a certain amount of chemical knowledge derived from my general reading, I suppose mainly confined to descriptions of the individual elements, and an awareness of the Periodic Table. However, in the chemistry lab, on either side of the teacher's dais, there were displayed two enigmatic representations of the entire subject. On the left was the mid-20th-century version of the Periodic Table, and on the right was the Electrochemical Series. The immediately intriguing fact was that the latter was incomplete. It had potassium at the top and oxygen at the bottom, and a couple of dozen others in between. I wondered “Where were the rest?” Or maybe even “Why don’t they want me to know where the rest are?” I knew that the fearsomely reactive fluorine (not listed) belonged below oxygen. Where were - say - the lanthanides? Were they fun elements like the alkali metals? Or were they just boring transition metals? I started reading - and thinking - about this a lot. Long gazing at the beautiful mandala that is the Periodic Table brings on a metaphysical understanding of the essential nature of Chemistry.
A superficial look at the Periodic Table probably forms the stumbling block that puts most people off chemistry. Clearly, learning chemistry - and being a chemist - consists of learning all the physical and chemical properties of an almost infinite number of compounds of each element, of which there are an intolerably large number. John Dalton introduced the idea of atoms in 1808 without affecting science at all. Natural philosophers just regarded atoms as an alchemical eccentricity. Before Mendeleev, scientists steeped in the ideas of the Age of Reason assumed that new elements (as opposed to atoms) would go on being discovered, ad infinitum. However, the first bit of good news in Mendeleev's Table is that there are only 85 elements sufficiently stable to exist and be chemically investigated. This is an immediate outcome of the fact that the set of elements is defined by column number and row number, both of which are integers. So there will never be discovered an element (say) between boron (5) and carbon (6).
Many of Mendeleev's contemporaries would have been horrified by such a suggestion. All known determined physical quantities were represented by floating point numbers, with the number of decimal places limited only by the precision of the determination. However, as with other great breakthroughs in science, Mendeleev's model was so beautiful that objections soon faded away. The integral nature of atoms which we would now call atomic number, was finally demonstrated by Moseley in 1914, in the form of the simple integer sequence of atomic X-ray spectra. What is more, Mendeleev's adoption of an integer model prefigured quantum theory and much of 20th century physics.
In a way, if the reality of atoms had not been proved in the early twentieth century, it would have made no difference to chemistry's philosophical position. It differs fundamentally from physics. Physics is fundamentally analytical in approach. It begins (as it did in our first-year Grammar School physics class) by measuring the "physical properties" of lumps of stuff. There then follows a process of subdivision in which the physical properties of the lump's components are measured, and their contributions to its bulk properties are explained, And so on, ad infinitum - to quarks and beyond. On the other hand - and this can't be emphasised too much - chemistry is fundamentally synthetic. It's about making things (Note 3).
At university, one of many day-one purchases was a box of Aldrich-Fieser molecular model parts. These could be assembled into small or large molecular models. This is the quintessence of chemistry. Chemistry begins with a box of Dalton's atoms - you stick them together and make molecules, firstly as a thought experiment, then you do the same thing in a test tube, and watch with delight as the intended product crystallises out (or not!) The atoms are the unchallengeable axiom at the start of the process.
Mendeleev's Table thus shows chemistry to be the study of a complex array of chemical combinations, underlain by a simple rule set. I was attracted by this complexity and by the possibility that the properties of as-yet undiscovered compounds could be predicted. All the more attractive was organic chemistry in which the atoms in use could be reduced to half a dozen elements, which yet could be arranged in an infinite variety of ways. By the time of my A-levels (1967), I was thoroughly committed to Chemistry as my academic subject.
Organic Chemistry
So much for history. I think it must have been in the sixth form that the class made a minor excursion into organic chemistry - along familiar lines. We fermented some sugar and made some ethanol. We oxidised it to acetaldehyde (ethanal) and to acetic (ethanoic) acid. We reacted ethanol and acetic acid to make ethyl acetate. I think we even did the classic determination of the equilibrium constant of this esterification reaction (it's four!)
At University, there was opportunity to learn practical chemistry to a high level. Inorganic chemistry teaching was rather second-rate, but organic and physical chemistry courses were excellent. Organic chemistry has an immediate appeal for the lover of complexity. Using only carbon, hydrogen and oxygen - with just occasionally a bit of nitrogen and sulphur - an infinite number of compounds can be prepared, with properties that can be adjusted to order. Another attraction is that all preparative chemistry - whether organic or inorganic, but predominantly organic - is basically cookery. Alternatively, all cookery is organic chemistry. It has always seemed to me incredible that anyone who claims to be "no good at cooking" could simultaneously claim to be a chemist. For me, acquiring the artistry of preparative organic chemistry was the most stimulating and satisfying part of the work. A second line of interest was in NMR spectrometry, which at the time was emerging as a workhorse of organic chemistry. NMR is pure quantum mechanics, completely beyond the scope of classical physics, but once analysed, NMR spectra yield a wealth of structural information on organic molecules, indicating subtle aspects of their geometry and the nature of the chemical bonds holding them together. I became a master of NMR spectrum interpretation (Note 4).
In the organic chemistry lab, we followed two great cookery books - written by two great hands-on practitioners - Louis Fieser's Organic Experiments and Arthur Vogel's Practical Organic Chemistry. These two seem like chalk and cheese - Fieser the stellar Harvard professor, and Vogel the Woolwich Polytechnic drudge, but I still treasure these books equally. Fieser takes you on a Star-Trek ride around the outer reaches of chemistry, while Vogel gives you thousands of quality-tested recipes using every organic technique - the Delia Smith of chemistry. You read a recipe and think "I could try this!" - and you can if you have a kitchen/lab and a few ingredients.
These two books, and their authors, embody a lot of what I love about chemistry. I've already mentioned John Dalton and Dmitri Mendeleev - both generalists - maybe renaissance men - eccentric and obsessive. Arthur Vogel was Head of Chemistry at Woolwich Polytechnic. I was recently (2023) reading an account of his life by John Benstead (who was one of Vogel's last pupils) in which it is said that he and his department prepared more than 1000 pure chemicals. I'm sure this is out by at least one order of magnitude, if only because, for every compound prepared, they also produced multiple derivatives suitable to characterise them by the cross-referencing process of old-time organic analysis. For each compound, and each derivative, they would measure melting point, boiling point, density, refractive index and so on. This contributed to the vast tables of physical properties of organic compounds that one finds in reference books. This, of course, you will say is mere "grunt work". But somebody has to do the grunt-work, and when you look up the physical properties of some obscure chemical, you had better hope that they were determined by people as proficient as these. The by-product of such work was books such as Practical Organic Chemistry, containing descriptions of stress-tested procedures that are authoritative and reliable.
Louis Fieser, on the other hand, could never be accused of grunt-work. The flamboyant professor was a fundamental research pioneer in the chemistry of steroids and of polycyclic aromatics in general. He had rock star status at Harvard, enhanced by his demonstrations of artistry in laboratory practise, elegant and virtuosic enough to be filmed. His book emphasises economy of equipment and materials "sold at five-and-ten-cent stores". And yet the book, designed as an introduction to organic chemistry for first-year undergraduates of Harvard quality, takes the student into arcane fields. On the cover of my copy is embossed the structure of hexaphenylbenzene - a propeller-shaped molecule of moderate interest that would never be synthesised except for pure fun. You get to make it by high temperature Diels-Alder reaction of tetraphenylcyclopentadienone and diphenylacetylene. Not materials in everybody's store cupboard - but you get to make them too. Tetraphenylcyclopentadienone is made by alkali-catalysed condensation of benzil (1,2-diphenylethane-1,2-dione) with dibenzyl ketone (1,3-diphenyl-2-propanone). Diphenylacetylene is made by dehydrobrominating stilbene dibromide (1,2-dibromo-1,2-diphenylethane) with alkali at high temperature. And yes, you get to make all those starting materials as well. At every point, the sheer beauty of these substances is emphasised.
It seems inevitable, given the above, that at University, I put most of my energy into preparative organic chemistry. Of course, there were other aspects of a degree in general chemistry. Organic chemistry teaching was first rate. Physical chemistry teaching was good. Inorganic chemistry teaching - perhaps influenced by my relative lack of interest - was bad. A favourite war-story of mine is the lack of any pedagogical value of the inorganic chemistry practical classes. Among the many practical modules on offer (although I didn't recognise it at the time) was a complete set of classical cement analyses - gravimetric determinations of calcium, magnesium, silicon, aluminium and iron. Plus sulfur as sulfur trioxide. The latter was particularly apposite. I still have the laboratory notebook in which these determinations were neatly reported. Much later, when I joined the cement industry, the SO3 determination was something one learned to do with great proficiency. Later still, involved in the arcane manufacture of sulfoaluminate cements, where sulfate levels are way outside the calibration range of the fast instrumental methods that replaced the classical analysis, I called upon my experience to deploy the classical method, with a demonstrated high level of precision and accuracy. However, back in the university lab, I was just given a cement sample and a set of instructions, which I duly followed and reported a result. The supervisor wrote on it "5/10 - result outside acceptable range". And that was it. What did I learn? Only that I wasn't very good at sulfate determinations. If I had had a summer job at a cement plant (but why would I?), I would have been OK. Because they would have taught me.
But physical chemistry practicals were great, with lots of challenging exercises in experimental technique, and plenty of time (or perhaps I should say overtime) to repeat and polish the process. One particularly beautiful experiment was the Swan Bands Analysis. I don't know if this was ever done elsewhere. The experiment was exquisitely simple, the apparatus consisting only of an old-fashioned goniometer spectroscope and a Bunsen burner. The Swan Bands are absorption lines in the visible spectrum of the inner part of the Bunsen flame. They derive from the π-π* electronic transition of the C2 molecule, convoluted with the vibration-rotation spectrum of the molecule to produce a fine structure. By carefully measuring the position of the lines, a number of fundamental properties of the molecule can be deduced. The rotational energy levels give the rotational moment of inertia of the molecule (and since its mass is known, this gives the bond length). The vibrational energy levels give the bond's elastic modulus, while its attenuation at higher energy levels give the bond dissociation energy. These all confirm that the molecule has a double bond. None of this can be derived without quantum theory. This simple molecule has a roughly parabolic energy profile. I later went on to investigate much more complex vibrational systems in quartic energy wells, using computed solutions of the Schrödinger equation - a process that tests desktop computers to their limits. Is the C2 molecule relevant? Well, the Swan band emission is a major contributor to flame radiational heat exchange in a cement kiln!
Then there was the organic practical course which, particularly in the third year, was magnificent, consisting of a large number of preparative processes. We all did Louis Fieser's Martius Yellow race (see, e.g. article). The race involves the seven-stage synthesis of the dye, including saving fair specimens of all the intermediates. Points are scored mainly for speed, but also for yield and purity of products. As the dye is very effective on human skin, points are deducted from students who end up looking like Homer Simpson. Since I believe in immersing myself in my work, I did lose points on that account, but I made some good Martius Yellow.
Organometallic chemistry
Throughout my undergraduate years, I developed an interest in organo-metallic chemistry, simply because of the extra dimension of complexity that it afforded. Furthermore, the introduction of electropositive metals - Li, Na, K, Mg, Al (Note 5) - to organic molecules produces highly reactive reagents that can be used in many synthesis processes.
All this was background which led to my investigating many organo-metallic processes after graduation, under the supervision of Colin Young. His previous students had been investigating the formation of organo-silicon free radicals by reaction with the alkali radical anions of polycyclic aromatic hydrocarbons.
My immediate predecessor had been attempting the reactions of the radical anion of pyrene. This was ill-advised, since it led to an intractable mixture of reaction products that were impossible to separate. His last attempt at a reaction that might work was to react the pyrene radical anion with carbon dioxide, forming carboxylic acids which were then reacted with diazomethane to produce the corresponding methyl esters. A tiny amount of pure product resulted (Note 6). My first job, on day one, was to identify this product from its 60 MHz NMR spectrum. I reported back, after a couple of hours' work, that it was 1,5-bis-carboxymethylpyrene. This attribution, which was greeted with a little scepticism, was subsequently confirmed by running the NMR at 220 MHz (Note 7), which makes the structure of the spectrum much simpler and more obvious.
I ought to explain the nature of organic radical ions. In unsaturated organic molecules, a π-bond results from placing two electrons in a bonding pπ orbital. For every such orbital there also exists an anti-bonding pπ* orbital, occupation of which is energetically unfavourable and undoes the bond. If the molecule contains a large array of conjugated π-bonds, the uppermost bonding orbital approaches closely below the level of energy neutrality, while the corresponding anti-bonding orbital is closely above it. Such a molecule can lose an electron from the uppermost bonding level, or acquire an electron in the lowest anti-bonding orbital, with a comparatively small energy penalty.
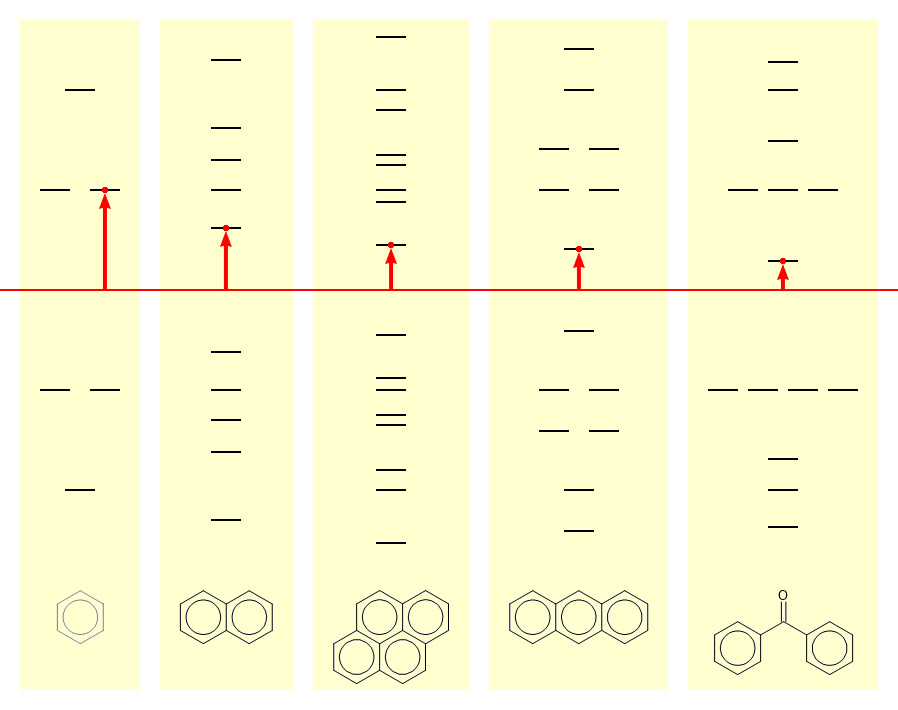
A relatively familiar example is the benzophenone molecule (Note 8). Dissolved in dry diethyl ether, it produces a colourless solution. Addition of some fine sodium wire results in a bright blue solution. The sodium transfers its spare electron to the lowest anti-bonding orbital of the benzophenone. The loss of the electron by sodium, and its acquisition by benzophenone are both in themselves energetically unfavourable, but the extra energy required is obtained by solvation of the sodium ion by the ether solvent.
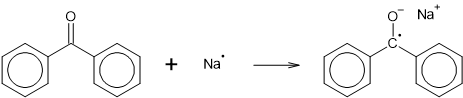
With its extra electron, the benzophenone is now an anion, and because the extra electron is un-paired, it is also a free radical. That is, it's a radical-anion. This species is stable as long as it is protected from air and moisture, but it is a powerful nucleophile, and reacts with water to produce benzhydrol.

With carbon dioxide, added as dry ice, it gives benzilic acid.

The pyrene compound was obtained from the pyrene radical anion, produced using sodium in tetrahydrofuran (THF) solution. THF is a more powerfully solvating aprotic solvent than diethyl ether. Reaction of this with carbon dioxide (in the form of dry ice) gives 1,5-dicarboxy-1,5-dihydropyrene. This was converted to 1,5-dicarboxymethyl-1,5-dihydropyrene by addition of diazomethane. Attempts to separate this from many other products by chromatography on activated alumina caused it to dehydrogenate.
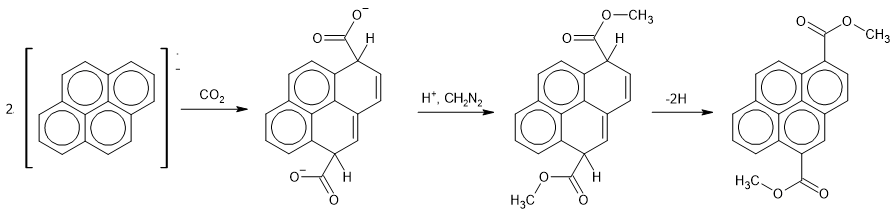
It was obvious that pyrene was not a suitable molecule to study because of the many possible reaction products. The previous researcher had used naphthalene. I tried making a number of interesting radical anions, and reacting them with chlorotriphenylsilane, including those of 1-methylnaphthalene, 2-methylnaphthalene and ferrocene. The latter results in formation of 1-triphenylsilylcyclopenta-2,4-diene. I decided eventually that anthracene, with a similar electron affinity to pyrene, and reaction taking place almost exclusively at the 9,10-positions, was a more straightforward proposition.
My next job was to make a supply of chlorotriphenylsilane, the previous supply having been depleted by the previous researchers. This material was not available commercially (or if it was, it commanded an exorbitant price). So I made phenylsodium from sodium and chlorobenzene in petroleum ether. This is one of many reactions conducted in dry, oxygen-free conditions: reagents and apparatus were all rigorously dried, and reactions were conducted under oxygen-free nitrogen, bubbled through benzophenone/sodium adduct solution - or even through liquid sodium/potassium eutectic. The phenylsodium solution was added to dichlorodiphenylsilane - readily available as a silicone precursor. On filtering, stripping the solvent and fractional distillation under vacuum, this gave chlorotriphenylsilane, m.p. 92-94°C.
Anthracene reacts with sodium in THF to produce a blue radical anion, and on addition of further sodium, a green di-anion. Using these, I made 9,10-bis-trimethylsilyl-9,10-dihydroanthracene and 9,10-bis-triphenylsilyl-9,10-dihydroanthracene. The common mechanism with the radical anion proceeds by:
- 1) electron transfer from the radical anion to the chlorosilane to produce a chloride anion and a silane free radical.

- 2) rapid reaction of the free radical with the hydrocarbon to produce an adduct free radical and
- 3) reaction of the latter with a second silane free radical.
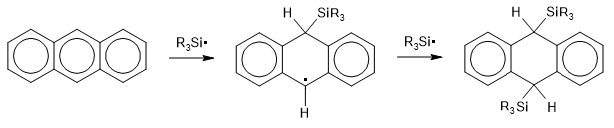
The "hard" nature of the silane free radicals is key here. They contrast greatly with their carbon-based equivalents. It's well known that the triphenylmethyl free radical is quite stable, and results when attempting to make the non-existent hexaphenylethane molecule. In the original (Gomberg, 1900) experiment, bromotriphenylmethane was reacted with zinc dust in benzene, producing a yellow-coloured solution containing (it turned out) triphenylmethyl free radicals. These fail to dimerise to hexaphenylethane because
- 1) the radical is stabilised by delocalisation of the extra electron over three benzene rings
- 2) the central bond of hexaphenylethane is unfeasibly long due to crowding.
The difference with the silicon analogue is striking. Not only does hexaphenyldisilane exist, but it is extremely stable - it can be distilled at atmospheric pressure at dull red heat without decomposition. In this case
- 1) the free radical is unable to delocalise because silicon d-orbitals fail to couple with phenyl p-orbitals
- 2) the Si-Si and Si-C bonds are naturally longer, relieving crowding.
The same effect is to be noticed in the dihydroanthracene compounds: 9-triphenylmethyl-9,10-dihydroanthracene is easily decomposed whereas 9-triphenylsilyl-9,10-dihydroanthracene is perfectly stable.
9,10-disubstituted-9,10-dihydroanthracenes have two possible geometric isomers - cis- and trans-.
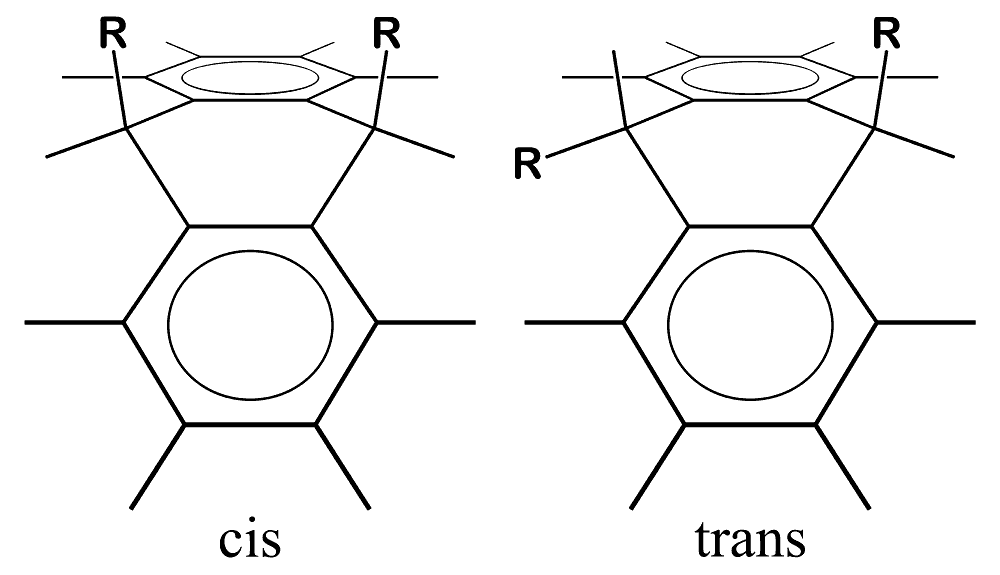
The question of which isomer was formed is associated with the question of the geometry of the intermediates. Using the above techniques, as well as several other preparative approaches, I attempted to produce a wide range of compounds of known geometry. One straightforward method was to add triphenylsilyllithium to anthracene, producing 9-lithio-10-triphenylsilyl-9,10-dihydroanthracene, which can then be reacted with a variety of halides RCl to produce 9-R-10-triphenylsilyl-9,10-dihydroanthracene. I did this for R = methyl, ethyl, tert-butyl, triphenylmethyl, trimethylsilyl and triphenylsilyl. Triphenylsilyllithium was made by cleaving hexaphenyldisilane (available in kilogram quantities in my lab) with lithium in THF. In order to restrict the geometry of the intermediates, similar di-anion reactions were performed with 9-methylanthracene and with 1,4-dimethylanthracene. As shown above, the dihydroanthracene molecule is folded, so that the 9- and 10- substituents have pseudo-equatorial and pseudo-axial positions. 1,4-dimethylanthracene discourages 9- or 10- substituents from assuming the pseudo-equatorial position by crowding. Both were home-made: 9-methylanthracene by Grignard methylation of anthrone, and 1,4-dimethylanthracene by Friedel-Crafts acylation of p-xylene with phthalic anhydride to form 1,4-dimethylanthraquinone followed by reduction with zinc.
A further set of specimen NMR spectra was obtained by preparing 9-substituted fluorenes. These define molecules which are more-or-less flat, whereas in 9,10-dihydroanthracenes, the central ring can be folded into a "boat" conformation. My questions were:
- 1) does an attacking free radical approach in an axial or equatorial position?
- 2) does the second free radical approach in such a way as to produce a cis- or a trans- product?
What geometry is in practice obtained?
NMR spectrometry in theory enables assignment of the geometry. I had prepared and characterised dozens of compounds, complete with NMR spectra. At this point, I made a fatal decision - I used as a source of assignment rules a paper which assigned diametrically incorrect conformations. This was based on what I would retrospectively see to be a nonsensical analogy with cyclohexane compounds, to say whether an axial proton resonated up-field or down-field from its equatorial neighbour. But it was a peer-reviewed paper in a well-respected journal and, in my naivety, I had thought that sufficient. I therefore continued for a period of several month incorrectly assigning the geometries of my molecules, and trying to rationalise them in my draft thesis.
Eventually I found a paper which assigned NMR lines correctly. On reading this, it was immediately and obviously correct. This plunged me into despair. I now realised that what I had been writing was nonsense. I would have to completely re-write, and I was practically out of time to do so. Shortly after that, I got a message from the head of department, John Meurig Thomas - naturally delivered not to my face but via my supervisor, Colin Young - that I should write up immediately or leave.
I left.
I could probably have retrieved the situation given high doses of antidepressants, but this just didn't seem feasible at the time. This marked the end of my career as an academic chemist. That period had been most definitely the time of my life. I had done more solid chemistry in eighteen months than most chemists do in a lifetime, mostly unsupervised, and had become the master of many techniques, both theoretical and practical. One very significant development was that I became a member of the Chemical Society. My sponsors were, I think, Colin Young and Alun Price.
It's worth describing the learned societies that existed in British chemistry at the time. What is today the Royal Society of Chemistry was then represented by several independent bodies:
- 1) The Chemical Society, founded in 1841, was for academics, and published the Journal of the Chemical Society as a means for its members to circulate their work.
- 2) The Society for Analytical Chemistry, founded in1874 as the Society of Public Analysts, was the professional institution for that subset of chemists.
- 3) The Royal Institute of Chemistry, founded in 1877, was the professional organisation, issuing its own qualifications, for salaried leaders of the industry.
- 4) The Faraday Society, founded in 1903, was the academic society specifically for physical chemistry, and published its own journals.
My membership of the Chemical Society enabled me to publish my work. One also got personal access to publications such as Chemistry in Britain, Quarterly Reviews, and at least one Journal - for me Perkin Transactions - in return for ongoing subscriptions. Thus, even after leaving academia, I was able to stay up-to-date with the latest developments in my subject.
After leaving, there followed a year in which I attempted to get work in various organic chemistry disciplines suited to my skills - always with another message from John Meurig Thomas - again received second- or third-hand - that he would see to it that I didn't get a decent job. Eventually I was recommended by a desperate Job Centre mentor to apply for an advertised job as "Relief Slurry Tester" at APCM's Sundon plant. By that stage, my self-esteem was sufficiently attenuated, that I would take anything. The Job Centre guy phoned them to find out if they would interview me. Back down the line came the decisive question - "Can he read a scale?" Yes, I replied.
Although the prospect of this job was depressing in the extreme, I received a small boost at the interview. I was interviewed by Jack Eagling, the Technical Manager, and Len Hillsdon, his deputy. Len became my great mentor during my formative years in the cement industry. They were, respectively, horrified and amazed that a graduate would be applying for this job. Len said "We need people like you". It was the nicest thing anyone had said to me in years. I started on Thursday 13 September 1973.
So I joined the cement industry - a creature from whose maw no-one escapes alive. The amount of chemical knowledge required in the cement industry is infinitesimal, but I was still able to bring some of my more general skills to bear - experimental design and the statistical basis of precision in measurement. I applied myself to projects such as the hopeless task of reducing slurry moisture at Sundon. I also became a geologist in getting an understanding of Sundon's very complex quarry.
With Len Hillsdon as Technical Manager, Sundon entered a short period prior to its closure in which the process of data gathering and reporting was revolutionised. As a general rule on cement plants, the data produced by various testers - who are virtually unskilled labourers - went through several stages of vetting before being released to the wide world in neat form, duly signed off by the Technical Manager. Len Hillsdon initiated a regime in which the final records were filled in by those who made the measurements. It is difficult to over-emphasise how different this was from normal practice as observed by me throughout my career at hundreds of cement plants world-wide. Data were routinely falsified. In some cement plants, original data were never published - all data were false. In some plants, falsification was just an "easy way out" for lazy quality management. In some plants, it was mandated from the highest levels of management. There is an urban legend that, when Ken Bezant (Technical Director) was required to sign a memo to Plant Managers saying they should desist from telling their Quality Managers to cook the books, he said "I hope no-one remembers when I was a Plant Manager". In later years, it always amused me to see plant problems being diagnosed by analysis of data which was undoubtedly 24-carat bollocks. However, during my first introduction to the cement industry at Sundon, all data was real, and as a naive trainee, I assumed that it was thus everywhere.
The Sundon plant shut down at the end of 1976. Frank Langcaster - another individual who was very influential in my development - had the unenviable task of implementing a half-arsed Statistical Process Control strategy for quality control in APCM's Northern Area. When the closure was announced, he said "Well, it was inevitable, wasn't it?" I rather envied his Olympian view of fate. It meant I had to move. It was put to me that I might want to take on the microscopy job at the Northern Area lab in Hull. In the end it was decided that I was too undesirable for a job in Area Office, and I was made an offer I couldn't refuse - to take over from Ron Mayman, the retiring Technical Assistant at the Humber plant. In losing the microscopy role, I dodged a bullet - the job was instead taken by Bob Blezard, recently redundant from West Thurrock, and that didn't end well, with the closure of the Northern Office a few years later.
At Humber, I was effectively No 3 and stand-in for Henry Smith, the Technical Manager, and Peter Ellis, his deputy. On day one, I made the naive mistake of reporting a set of low strength data, as determined. All hell broke loose. Frank Langcaster phoned in a state of panic. My calm explanation of the cause seemed to make matters worse. Henry Smith called me into his office. He showed me the ledger of "official" test data. "See", he says, running his fingers down the columns, "we just don't get numbers like that". I was puzzled - clearly we do! Gradually I was introduced to the way things were done at Humber. All data was falsified. Not a single genuine number was ever published. This was partly down to Frank Langcaster's control system. Strengths were expected (i.e. required) to remain close to the target level. 1.5 MPa below the target level (regardless of variability) was the "Early Warning Level". If two successive results fell below "Early Warning Level", a notification of a quality change was issued to customers. As Henry Smith explained to me, "we don't get Early Warnings". On one memorable occasion, seventeen successive Humber strength results below Early Warning were obtained, bottoming out 6 MPa below target. All these were reported as above Early Warning Level. And just in case this should be regarded purely as an "inside job", I also had it impressed upon me by Frank Langcaster that it was important to "be discrete".
Although some aspects of Humber were fun, I could not long stand the totally demoralised atmosphere of the place, and I applied for the Deputy Technical Manager's job at Shoreham when it came up. Not having been there, I asked Len Hillsdon what he thought of the plant. "A bit sleazy", he said. By contrast with Humber, that sounded pretty good.
Mark Gaskell, Shoreham's Technical Manager, had clearly been in contact with Henry Smith for references. On the day I started in February 1979, he said "the first thing you need to know is that, regarding strength data, we don't like to frighten people". You will perhaps agree with me that it's not so much the complete lack of professional ethics and moral fibre that horrifies - it's the mealy-mouthed way in which it is expressed.
This brings my narrative arc to its conclusion. In 1980, the four constituent organisations unified to form the Royal Society of Chemistry. As a professional organisation it had a written Code of Conduct which all members had to sign. Reading through it, I realised that, as an employee of an industry in which professional ethics are left at the gate, I could not in conscience sign. So I let my subscription lapse, and my involvement with the subject I loved with a great passion lapsed with it. Do I resent this? Well, should I?
I could go on to describe the ensuing thirty years I spent in the cement industry, but as it is a more-or-less chemistry-free zone, such an account doesn't belong here.